Effect of pH on MnOx/GACcatal yzed ozonation for degradation
论文类型 | 技术与工程 | 发表日期 | 2005-06-01 |
作者 | 马军 | ||
摘要 | School of Municipal and Environmental Engineering, Harbin Institute of Technology, P.O. Box 2627, 202 Haihe Road, Harbin 150090, PR China Received 23 March 2004; received in revised form 6 September 2004; accepted |
School of Municipal and Environmental Engineering, Harbin Institute of Technology, P.O. Box 2627,
202 Haihe Road, Harbin 150090, PR China
Received 23 March 2004; received in revised form 6 September 2004; accepted 11 November 2004
Abstract
The effect of pH on MnOx/GACheterogeneous catalytic ozonation was investigated. Nitrobenzene was used as a model refractory organic pollutant. It was found that in MnOx/GACcatalytic ozonation, the degradation efficiency of nitrobenzene was higher under low pH conditions (pH 2.74–3.52) than that under high pH conditions (pH 6.72–9.61). This result was different from the case of ozonation alone, in which higher pH had positive effect on the degradation of nitrobenzene due to the formation of hydroxyl radical. In the presence of MnOx/GACcatalyst, ozone decomposition was accelerated, and higher pH condition favored ozone decomposition. It was assumed that hydroxyl radicals might not be the dominating active species in the catalytic oxidation, for the presence of t-butanol did not have any influence on MnOx/GACheterogeneous catalytic ozonation. Adsorption of organic micropollutants on MnOx/GACcatalyst was an important step and would have direct influence on the effectiveness of catalytic oxidation. It was assumed that the organic pollutants might be further decomposed on the surface of catalyst.r 2005 Elsevier Ltd. All rights reserved.
Keywords: pH; MnOx/GACheterogene ous catalytic ozonation; Ozone decomposition; Adsorption; Nitrobenzene; t-butanol
1. Introduction
Heterogeneous catalytic ozonation has received increasing attention in recent years due to its potentially higher effectiveness in the degradation of refractory organic pollutants and lower negative effect on water quality. The ozonation of refractory organic pollutants
could be enhanced by the presence of solid catalytic materials. Al-Hayek et al. (1989) found that catalytic ozonation of phenol in the presence of Fe3+/Al2O3 had an increase of TOCremoval than ozonation alone under the same reaction condition. The authors suggested that either a formation of free radicals or an increase of nucleophilic sites of adsorbed molecules was responsible for the enhanced degradation of refractory organic micropollutants. Paillard et al. (1991) reported that TiO2-catalyzed ozonation was more efficient for the
egradation of humic acid than ozone alone. Paillard et al. (1991) suggested the TiO2-catalyzed ozonation did not follow a radical mechanism, since the presence of bicarbonate, a typical radical scavenger, was not found to have any influence on TiO2-catalytic ozonation. Bhat and Gurol (1995) studied the ozonation of chlorobenzene in the presence of goethite and found that catalytic ozonation was moreeffective than ozone oxidation alone. In recent years, manganese-catalyzed oxidation has received intensive investigations. Andreozzi et al. (1996, 1998, 2000) studied the ozonation of oxalic, pyruvic and glyoxalic acid in the presence of manganese salts and manganese dioxide. Ma and Graham (1997) demonstrated that the oxidation of atrazine by ozone was greatly enhanced in the presence of the colloidal
form of hydrous manganese oxides. The effects of humic substances, radical scavengers on the Mn-catalyzed ozonation were studied (Ma and Graham, 1999, 2000). In a recent investigation, Ma et al. (2004) supported manganese oxides (MnOx) on granular active carbon (GAC) to enhance the oxidation of nitrobenzene by ozone. The preliminary experimental results showed that the prepared catalyst was very effective for accelerating the degradation of refractory organic pollutants. In this paper, the effect of pH on MnOx/GAC catalytic ozonation was studied. It is well known that pH is an important factor for ozonation, since it affects ozone decomposition. Ozonation at different pH ranges follows different reaction mechanisms. In the case of MnOx/GACcatalyzed ozonation, pH may both affect the ozone decomposition and the surface property of the catalyst. Thus, it is necessary to study the effect of pH on heterogeneous catalytic ozonation, and this may be
helpful for further understanding the mechanism of MnOx/GACcatalyzed ozonation.
2. Materials and methods
2.1. Preparation and characterization of MnOx/GAC catalyst
The catalyst was prepared by supporting MnOx on GACby using the impregnation method. GAC(20?0 mesh in size, Taiyuan Activated Carbon Production Plant, China) was repeatedly rinsed by distilled water and dried at 105 1C. Then 5 g GAC was impreganated with 100mL 0.1 mol/L potassium permanganate solution (KMnO4) and constantly shaken for hours at room temperature. The prepared MnOx/GACcatalyst was
thoroughly rinsed with organic-free water before use. MnOx/GACcatalyst was characterized by X-ray power diffraction (XRD), X-ray fluorescence spectrometry (XRF), specific surface area and pHzpc. The XRD analysis was performed with a D/max-r B diffractometer, at 40 kV, 30mA (1200W) by using nickel-filtered Cu Ka radiation. The results indicated that the supported MnOx on GACwas amorphous and non-stoichiometric. The elemental analysis of the solid catalyst was carried out by XRF (XRF-1700, 40 kV70 mA, 40 s. Integration, fixed monochromatic, Shimadzu, Japan). The results indicated that the catalyst contained 10.8% (weight basis ratio) impregnated MnOx. The physical property of catalyst was determined by N2 adsorption at 77.4K with a high-speed automated BET analysis instrument (Nova4000, America), which can measure the amount of adsorption from 1.328104 kPa with an accuracy of 0.25% of the reading pressure values. The amount of adsorption in the low-pressure range was calculated after correction for pressure due to the thermal transpiration effect. The samples were degassed at 200 1Cbelow 1MPa for 4 h in the nitrogen environment before determination. The results are summarized in Table 1. The pHzpc of the MnOx/GACcatalyst was 7.60 (determined by the method proposed by Mullet et al. (1999)).
2.2. Materials
Model polluted water was prepared by spiking nitrobenzene (Beijing Chem. Ltd.) in distilled water. Nitrobenzene is a typical refractory organic micropollutant, which is difficult to be oxidized by ozone alone (k ?0:09 0:02M1 s1; Hoigne?and Bader, 1983); while it could be easily oxidized by hydroxyl radical with the reaction rate constant of 2.2108M1 s1 (Hoigne?and Bader, 1976). To a certain extent,
nitrobenzene could be used as an indicator of radical chain reaction. The initial concentration of nitrobenzene was about 36 mg/L. The pH was adjusted with perchloric acid and sodium hydroxide. The t-butanol (TBA) (Beijing Chem. Ltd.) was diluted to specific concentrations before use. Other reagents such as potassium permanganate, sodium thiosulphate, potassium iodide, potassium indigo trisulfonate,
etc., were all reagent grades and were used without further purification. All glassware was soaked in chromic acid, then rinsed with tap water and distilled water.
2.3. Ozonation procedure
The ozonation tests were conducted in a bench scale ozonation system, which consists of an ozone generator (DHX-I, Harbin Jiujiu Electrochemistry Technology Co. Ltd. China), ozone reactor column (600mm in height and 40mm in diameter) of 0.7L volume, and a peristaltic pump (LEAD-1, Baoding Lange Pump Co. Ltd., China) for feeding the model water into the reactor. A schematic diagram of the ozonation system is shown in Fig. 1. Ozone was produced from an ordinary grade air and fed into the ozone contact column through a porous glass frit at the base of the column, which enables small bubbles to be produced. The residual ozone in the off-gas was adsorbed by KI solution and analyzed by using the standard method.
Before conducting the test, the catalyst was put into the reaction column. The experiment was performed in continuous (catalytic ozonation process) or semicontinuous (ozonation process) flow mode. In the continuous flow mode the flow rate of water is 0.075 L/min, the hydraulic retention time (HRT) was 8 min. In the case of ozonation alone, 0.6L of model water was fed into the reactor column first and ozonated for 8 min, and then the samples were taken out through the outlet. The oxidation reaction was quenched by adding a small amount of sodium thiosulphate. In the adsorption test, air was fed into the reactor column under the same operation condition with ozonation. The test of ozone decomposition was carried out as follows: Firstly, ozone was introduced into a 0.6L distilled water without pH adjustment, then a certain amount of catalyst was added rapidly, and the pH was adjusted by perchloric acid or NaOH. At the same time the magnetic stirrer was switched and the samples were withdrawn at different intervals to determine the residual ozone concentration.
2.4. Analysis
Concentration of nitrobenzene was analyzed by using a SP-502 Gas Chromatograph (GC) equipped with an electron capture detector (Lu Nan Instrumental Factory,Shandong, China). The GC was connected with an on-line GCworkstation, which recorded the data and GCspectra. The chromatographic conditions were as follows: column temperature 160 1C; detector temperature 200 1C; injector temperature 200 1C; carrier gas was nitrogen. The pH was recorded by pHS-2Canalyzer (Lei Ci Instrumental Factory, Shanghai, China). The ozone concentration in the liquid was determined by the Karman-Indigo method (Bader and Hoigne?, 1981).
3. Results
As mentioned above, in a heterogeneous catalytic ozonation system, pH has two main direct influences on the oxidation, i.e., ozone decomposition and the surface property of solid catalyst. On one hand, it is well known that ozone decomposition usually has a high dependence on pH. As shown in Fig. 2, ozone decomposition into active species is catalyzed by OH ion, and the primary radicals such as HOd is formed, which reacts with the organics in a non-selective mode and exhibits high reaction rate constants with the organics (Staehelin and Hoigne?, 1982). It is also reported that OH is a rather poor initiator of aqueous phase radical chain reaction that accelerates the transformation of ozone. It is reported that OH ion exhibits an especially low reaction-rate constant (k ?70 7M1 s1) with ozone, and is only relevant in very pure water (Staehelin and Hoigne?, 1982). Ozone decomposition is also affected by pH, which is related to the equilibrium concentrations of the
dissociated forms of inorganic carbons in aqueous matrix (HCO3/CO3 2, pKa (HCO3/CO3 2) ?10.3). As shown in Eqs. (1) and (2), carbonate is a much stronger scavenger than bicarbonate. HCO3 ?HOd !H2O ?CO3 d; k ?1:5 107LM1 s1餒oign 磂 and Bader; 1976? (1)
CO3 ?HOd! OH ?CO3 d; k ?4:2 108LM1 s1餗asten and Hoign 磂; 1992?
(2) On the other hand, in heterogeneous catalytic system, pH could affect the surface property of metal oxides (MnOx), which are covered by surface hydroxyl groups in the presence of water. Under different pH conditions, there exists proton transference on the surface of metal
oxides (Stumm and Morgan, 1981). It is shown in Eqs. (3) and (4) that with the increase of pH, the
MeOH2
decreases and more MeO are produced. These species have associations with the adsorption of organics on the catalyst (Liu and Tang, 2000). Up till now, no information has been obtained about the specific interactions of ozone with these species on the surface of metal oxides, but it could be deduced that these species may play an important role for ozone decomposition in heterogeneous catalytic process. RMeOH?
RMeOH ?H? (3) RMeOH MeO ?H? (4)
3.1. Effect of pH on ozonation
The effect of pH on ozonation was studied in semicontinuous mode. As shown in Fig. 3, in acidic and weakly acidic conditions, the ozone molecule itself was one of the main reactive species, so the degradation of nitrobenzene exhibited low efficiency. After the pH was
increased over 6.40, especially higher than 8.30, the degradation efficiency of nitrobenzene began to rise obviously. When pH increased from 8.30 to 10.30, the degradation efficiency increased from 30% to 86%. This was due to the OH ions that functioned as the initiator of radical chain reaction to form hydroxyl radicals, which have high reaction reactivity towards nitrobenzene (k ?2:2 108M1 s1; Hoigne?and Bader, 1976). From Fig. 3, it was also noted that the optimum pH for ozone oxidation of nitrobenzene is 10.30 in this test. At much higher pH, the degradation efficiency decreased. The negative effect should be due to the scavenging potential of carbonate or the interactions between hydroxyl radicals (Gottschalk et al., 2000). Because the solution was prepared with distilled water, the concentration of inorganic carbon was low and the inhibiting impact of carbonate on the oxidation could be
3.2. Effect of pH on MnOx/GAC catalyzed ozonation
The tests were originally planned and carried out in semicontinuous flow mode. However, it was found that except for the initial pH which was adjusted in strong acid region (o3), the pH in effluent (pH about 9) was much higher than that of the influent. In catalyst preparation process, such reaction occurs:
MnO4 t C t H2O !MnOx t CO t OH: (6)
These OH ions may be physically adsorbed on the surface of the catalyst, and they are also easily desorbed into the solution. Whether these adsorbed and desorbed OH ions contribute to the catalyst activity is very interesting to investigate. So the test was planned to
measure the effluent pH continuously. Also, for the heterogeneous catalytic ozonation, long-time continuous tests could give a deeper understanding of pH influence on MnOx/GACcatalyzed ozonation. In the tests, the solution with influent pH 2.65 was adjusted by perchloric acid, and the solution with influent pH 5.98 was not adjusted with acid or base. The experimental results are shown in Tables 2 and 3
and Fig. 4. From Table 2, it is seen that when the influent pH is 2.65, the effluent pH values were slightly changed during the catalytic reaction (2.74–3.52). When the influent pH is 5.98, as illustrated in Table 3, within the initial 15 min of the reaction, the pH of the effluent increased from 5.98 to 9.51–9.61. As the reaction went on, the pH in the effluent gradually decreased. When the reaction time was extended up to 230 min, the effluent.
pH decreased to about 6.72. In contrast to this pH change, it was found from Fig. 4 that the degradation efficiency of nitrobenzene did not change with the variation of pH in the solution. From the experimental results, it was expected that in MnOx/GACheterogeneous catalytic ozonation, the surface property of metal oxides, rather than OH ions in the solution, was the dominating factor influencing the catalytic activity. As shown in Fig. 4, it was obvious that in catalytic ozonation, the solutions with pH 2.74–3.52 (acidic condition) had much higher degradation effectiveness of nitrobenzene than the solutions with pH 6.72–9.61 (neutral pH and weak basic condition). Under acidic
condition (pH 2.74–3.52), the degradation efficiency of nitrobenzene was as high as 60%, while in the solutions with pH 6.72–9.61, the degradation efficiency of nitrobenzene was about 50%. Though the tests of the effect of pH on ozonation and catalytic ozonation were performed in different modes (semicontinuous and continuous modes), by comparing the results shown in Figs. 3 and 4, consistent with
previous research results (Ma and Sui, 2002), the MnOx/ GACcatalytic ozonation process is superior to ozonation for the micropollutants degradation.
4. Discussion
In the catalytic ozonation, the understanding of how solid catalyst influences ozone decomposition and the organics adsorption in different pH ranges is helpful for studying the reaction mechanism. As shown in Fig. 5, the presence of MnOx/GACobviously accelerated the decomposition of ozone, and the ozone decomposed faster in pH 5.60 than in pH 3.60. It is also noted that in the presence of catalyst, the decomposition rates at pH
3.60 and 5.60 are similar to the case when pH is 9.10 in the absence of catalyst. Whether hydroxyl radical is also produced in heterogeneous catalytic ozonation is the key
point for understanding the reaction mechanism. Until now, no clear conclusions have been drawn out on catalytic oxidation mechanism. On one hand, though having different postulations about how the initiation processes take place, a majority of authors assumed that the generation of hydroxyl radical due to ozone decomposition at the solid surface was mainly responsible for the improvement of catalytic ozonation (Ma and Graham, 1999; Cooper and Burch, 1999; Lima Hyung-Nam et al., 2002). Some investigators had different views, for they found that the presence of bicarbonate did not have any impact on some heterogeneous catalytic ozonation processes (Paillard et al.,
1991; Logemann and Annee, 1997). In order to evaluate if the MnOx/GACcatalyzed ozonation follows the hydroxyl radical reaction mechanism,
the effect of TBA on the catalytic oxidation was investigated. It is known that TBA is a strong hydroxyl radical scavenger which has the reaction rate constant of 6108M1 s1 with HOd (Buxton et al., 1988) and 3103M1 s1 with ozone (Hoigne′ and Bader, 1983). The TBA was added at 48, 100, and 202 min in influent pH 2.65, and 50, 55,100, 230 min in solutions with an influent pH of 5.98. If the catalytic oxidation follows hydroxyl radical reaction mechanism, the presence of 25 mg/L TBA would rapidly consume HOd?and have a negative impact on the degradation of nitrobenzene.Fig. 6 illustrates the effect of TBA on the oxidation of nitrobenzene by ozone alone in pH 8.32 and 11.80. It was seen that the addition of TBA obviously inhibited the degradation of nitrobenzene. However, it is shown in Figs. 7 and 8 that TBA did not have any inhibition on catalytic ozonation under the investigated pH conditions; the degradation efficiency of nitrobenzene was not
influenced in the presence of tert-butanol. From the experimental results, it may be assumed that hydroxyl radicals might not be the dominating active species in the catalytic MnOx/GACozonation process. The effect of pH on the adsorption of nitrobenzene is shown in Fig. 9. Under the two investigated influent pH values, the difference of adsorption efficiency of nitrobenzene was about 20%. It is obvious that low pH condition benefited for the adsorption of nitrobenzene on the catalyst. This result was consistent with the effect of pH on the catalytic ozonation. Then another question about the role of the catalyst is brought forward. Does the catalyst act more like an adsorbent
in the heterogeneous catalytic process? To clarify this question, continuous flow tests for longer time (hundreds of hours) were performed to compare the different degradation effectiveness of catalytic ozonation and adsorption. It is observed from Fig. 10 that, with the
extension of operation time, the adsorption efficiency of
nitrobenzene on MnOx/GACdecreased, and finally in about 400 h, the catalyst reached its adsorption capacity.MnOx/GACcatalyzed ozonation exhibited high degradation efficiency for nitrobenzene in the continuous tests. From the results it is not difficult to infer that in
MnOx/GACcatalyzed ozonation, the adsorption of organic micropollutants on catalyst is an important step, which would have direct influence on the effectiveness of heterogeneous catalytic oxidation; and the organic pollutants may be further decomposed on the surface
of catalyst.
5. Conclusions
Continuous flow experiments were performed to study the effect of pH on MnOx/GACcatalyzed ozonation, and the following conclusions could be made:
1. In the MnOx/GACcatalyzed ozonation, the oxidation efficiency of nitrobenzene was higher at low pH (pH 2.74–3.52) than that at high pH conditions (6.72–9.61). This result was different from the case of ozone oxidation alone.
2. In the presence of MnOx /GACcatalyst, ozone decomposition was accelerated, and higher pH was beneficial to ozone decomposition. It is assumed that hydroxyl radicals might not be the dominating active species in the catalytic oxidation, for the presence of t -butanol had less influence on MnOx/GACcatalyzed ozonation.
3. In MnOx /GACcatalyzed ozonation, the adsorption of organic micropollutants on catalyst was an important step, which would have a direct influence on the catalytic effectiveness of the heterogeneous catalytic system; it is also assumed that the organic pollutants would be further decomposed on the surface of catalyst. Acknowledgements The support from the National Natural Science Foundation of China (NSFC, Project 50378028) is greatly appreciated.
References
Al-Hayek, N., Legube, B., Dore′ , M., 1989. Fe3+/Al2O3- catalysed ozonation of phenol and its ozonation byproduct. Environ. Technol. Lett. 10, 415–425.
Andreozzi, R., Caprio, V., Insola, A., Marotta, R., Tufano, V., 1996. The use of manganese dioxide as a heterogeneous catalyst for oxalic acid ozonation in aqueous solution. Appl. Catal. A: General 138, 75–81. Andreozzi, R., Caprio, V., Insola, A., Marotta, R., Tufano, V.,
1998. The ozonation of pyruvic acid in aqueous solutions catalyzed by suspended and dissolved manganese. Water Res. 32 (5), 1492–1496.
Andreozzi, R., Marotta, R., Sanchirico, R., 2000. Manganesecatalysed ozonation of glyoxalic acid in aqueous solutions. J. Chem. Biotechnol. 75, 59–65.
Bader, H., Hoigne′ , J., 1981. Determination of ozone in water by the indigo method. Water Res. 15, 449–456. Bhat, N., Gurol, M.D., 1995. Oxidation of chlorobenzene by ozone and heterogeneous catalytic ozonation. 27th Industrial Waste Mid-Atlantic Conference, Bethlehem, PA, USA, July, p. 371.
Buxton, G.V., Greenstock, C.L., Helman, W.P., Ross, W.P., 1988. Critical review of rate constants for reactions of hydrated electrons, hydrogen atoms and hydroxyl radicals in aqueous solution. J. Phys. Chem. Ref. Data 17, 513–886. Cooper, C., Burch, R., 1999. An investigation of catalytic ozonation for the oxidation of halocarbons in drinking water preparation. Water Res. 33, 3695–3700. Gottschalk, C., Libra, J.A., Saupe, A., 2000. Ozoantion of Water and Wastewater. Wiley-VCH Verlag GmbH, D- 69469 Weinheim, p. 13. Hoigne′ , J., Bader, H., 1976. The role of hydroxyl radical reactions in ozonation process in aqueous solutions. Water Res. 10, 377–386.
Hoigne′ , J., Bader, H., 1983. Rate constants of reactions of ozone with organic and inorganic compounds in water-I: non-dissociating organic compounds. Water Res. 17 (2), 173–183.
Lima Hyung-Nam, Choib Hechul, Hwangc Tae-Moon, Kangc Joon-Wun, 2002. Characterization of ozone decomposition in a soil slurry: kinetics and mechanism. Water Res. 36, 219–229.
Liu Ruixia, Tang Hongxiao, 2000. Oxidative decolorization of direct light red f3b dye at natural manganese mineral surface. Water Res. 34 (16), 4029–4035.
Logemann, F.P., Annee, J.H.J., 1997. Water treatment with a fixed bed catalytic ozonation process. Water Sci. Technol. 35, 353–360.
Ma, J., Graham, N.J.D., 1997. Preliminary investigation of manganese-catalyzed ozonation for the destruction of atrazine. Ozone Sci. Eng. 19 (3), 227–240.
Ma, J., Graham, N.J.D., 1999. Degradation of atrazine by manganese-catalysed ozonation—influence of humic substances. Water Res. 33 (3), 785–793.
Ma, J., Graham, N.J.D., 2000. Degradation of atrazine by manganese catalysed ozonation—influence of radical scavengers. Water Res. 34 (15), 3822–3828.
Ma, J., Sui, M. H., 2002. Degradation of refractory organic pollutants by catalytic ozonation. Mn-modified activated carbon as catalyst. Advances in Ozone Science and Engineering: Environmental Processes and Technological Applications. 15–16 April, Hongkong, 185–192.
Ma, J., Sui, M.H., Chen, Z.L., Wang, L.N., 2004. Degradation of refractory organic pollutants by catalytic ozonationactivated carbon and Mn-loaded activated carbon as catalysts. Ozone Sci. Eng. 1, 1–9.
Masten, S.J., Hoigne′ , J., 1992. Comparison of ozone and hydroxyl radicals-induced oxidation of chlorinated hydrocarbons
in water. Ozone Sci. Eng. 14, 197–214.
Mullet, M., Fievet, P., Szymezyk, A., Foissy, A., Reggiani, F.C., Pagetti, J., 1999. A simple and accurate determination of the point of zero charge of ceramic membranes. Desalination 121, 41–48.
Paillard, H., Dore′ , M., Bourbigot, M.M., 1991. Prospects concerning applications of catalytic ozonation in drinking water treatment. The 10th Ozone World Congress Proceedings, Monaco, March, pp. 313–329.
Staehelin, J., Hoigne′ , J., 1982. Decomposition of ozone in water: rate of initiation by hydroxide ions and hydrogen peroxide. Environ. Sci. Technol. 16, 676–681.
Stumm, W., Morgan, J.J., 1981. Aquatic chemistry: chemical equilibria and rates in natural waters, 3rd ed. Wiley, NewYork, pp. 516–608.
202 Haihe Road, Harbin 150090, PR China
Received 23 March 2004; received in revised form 6 September 2004; accepted 11 November 2004
Abstract
The effect of pH on MnOx/GACheterogeneous catalytic ozonation was investigated. Nitrobenzene was used as a model refractory organic pollutant. It was found that in MnOx/GACcatalytic ozonation, the degradation efficiency of nitrobenzene was higher under low pH conditions (pH 2.74–3.52) than that under high pH conditions (pH 6.72–9.61). This result was different from the case of ozonation alone, in which higher pH had positive effect on the degradation of nitrobenzene due to the formation of hydroxyl radical. In the presence of MnOx/GACcatalyst, ozone decomposition was accelerated, and higher pH condition favored ozone decomposition. It was assumed that hydroxyl radicals might not be the dominating active species in the catalytic oxidation, for the presence of t-butanol did not have any influence on MnOx/GACheterogeneous catalytic ozonation. Adsorption of organic micropollutants on MnOx/GACcatalyst was an important step and would have direct influence on the effectiveness of catalytic oxidation. It was assumed that the organic pollutants might be further decomposed on the surface of catalyst.r 2005 Elsevier Ltd. All rights reserved.
Keywords: pH; MnOx/GACheterogene ous catalytic ozonation; Ozone decomposition; Adsorption; Nitrobenzene; t-butanol
1. Introduction
Heterogeneous catalytic ozonation has received increasing attention in recent years due to its potentially higher effectiveness in the degradation of refractory organic pollutants and lower negative effect on water quality. The ozonation of refractory organic pollutants
could be enhanced by the presence of solid catalytic materials. Al-Hayek et al. (1989) found that catalytic ozonation of phenol in the presence of Fe3+/Al2O3 had an increase of TOCremoval than ozonation alone under the same reaction condition. The authors suggested that either a formation of free radicals or an increase of nucleophilic sites of adsorbed molecules was responsible for the enhanced degradation of refractory organic micropollutants. Paillard et al. (1991) reported that TiO2-catalyzed ozonation was more efficient for the
egradation of humic acid than ozone alone. Paillard et al. (1991) suggested the TiO2-catalyzed ozonation did not follow a radical mechanism, since the presence of bicarbonate, a typical radical scavenger, was not found to have any influence on TiO2-catalytic ozonation. Bhat and Gurol (1995) studied the ozonation of chlorobenzene in the presence of goethite and found that catalytic ozonation was moreeffective than ozone oxidation alone. In recent years, manganese-catalyzed oxidation has received intensive investigations. Andreozzi et al. (1996, 1998, 2000) studied the ozonation of oxalic, pyruvic and glyoxalic acid in the presence of manganese salts and manganese dioxide. Ma and Graham (1997) demonstrated that the oxidation of atrazine by ozone was greatly enhanced in the presence of the colloidal
form of hydrous manganese oxides. The effects of humic substances, radical scavengers on the Mn-catalyzed ozonation were studied (Ma and Graham, 1999, 2000). In a recent investigation, Ma et al. (2004) supported manganese oxides (MnOx) on granular active carbon (GAC) to enhance the oxidation of nitrobenzene by ozone. The preliminary experimental results showed that the prepared catalyst was very effective for accelerating the degradation of refractory organic pollutants. In this paper, the effect of pH on MnOx/GAC catalytic ozonation was studied. It is well known that pH is an important factor for ozonation, since it affects ozone decomposition. Ozonation at different pH ranges follows different reaction mechanisms. In the case of MnOx/GACcatalyzed ozonation, pH may both affect the ozone decomposition and the surface property of the catalyst. Thus, it is necessary to study the effect of pH on heterogeneous catalytic ozonation, and this may be
helpful for further understanding the mechanism of MnOx/GACcatalyzed ozonation.
2. Materials and methods
2.1. Preparation and characterization of MnOx/GAC catalyst
The catalyst was prepared by supporting MnOx on GACby using the impregnation method. GAC(20?0 mesh in size, Taiyuan Activated Carbon Production Plant, China) was repeatedly rinsed by distilled water and dried at 105 1C. Then 5 g GAC was impreganated with 100mL 0.1 mol/L potassium permanganate solution (KMnO4) and constantly shaken for hours at room temperature. The prepared MnOx/GACcatalyst was
thoroughly rinsed with organic-free water before use. MnOx/GACcatalyst was characterized by X-ray power diffraction (XRD), X-ray fluorescence spectrometry (XRF), specific surface area and pHzpc. The XRD analysis was performed with a D/max-r B diffractometer, at 40 kV, 30mA (1200W) by using nickel-filtered Cu Ka radiation. The results indicated that the supported MnOx on GACwas amorphous and non-stoichiometric. The elemental analysis of the solid catalyst was carried out by XRF (XRF-1700, 40 kV70 mA, 40 s. Integration, fixed monochromatic, Shimadzu, Japan). The results indicated that the catalyst contained 10.8% (weight basis ratio) impregnated MnOx. The physical property of catalyst was determined by N2 adsorption at 77.4K with a high-speed automated BET analysis instrument (Nova4000, America), which can measure the amount of adsorption from 1.328104 kPa with an accuracy of 0.25% of the reading pressure values. The amount of adsorption in the low-pressure range was calculated after correction for pressure due to the thermal transpiration effect. The samples were degassed at 200 1Cbelow 1MPa for 4 h in the nitrogen environment before determination. The results are summarized in Table 1. The pHzpc of the MnOx/GACcatalyst was 7.60 (determined by the method proposed by Mullet et al. (1999)).
2.2. Materials
Model polluted water was prepared by spiking nitrobenzene (Beijing Chem. Ltd.) in distilled water. Nitrobenzene is a typical refractory organic micropollutant, which is difficult to be oxidized by ozone alone (k ?0:09 0:02M1 s1; Hoigne?and Bader, 1983); while it could be easily oxidized by hydroxyl radical with the reaction rate constant of 2.2108M1 s1 (Hoigne?and Bader, 1976). To a certain extent,
nitrobenzene could be used as an indicator of radical chain reaction. The initial concentration of nitrobenzene was about 36 mg/L. The pH was adjusted with perchloric acid and sodium hydroxide. The t-butanol (TBA) (Beijing Chem. Ltd.) was diluted to specific concentrations before use. Other reagents such as potassium permanganate, sodium thiosulphate, potassium iodide, potassium indigo trisulfonate,
etc., were all reagent grades and were used without further purification. All glassware was soaked in chromic acid, then rinsed with tap water and distilled water.
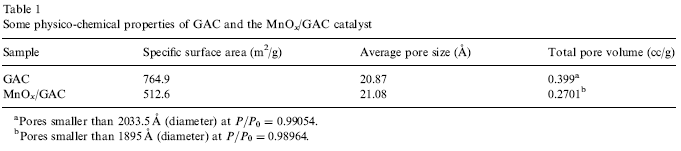
2.3. Ozonation procedure
The ozonation tests were conducted in a bench scale ozonation system, which consists of an ozone generator (DHX-I, Harbin Jiujiu Electrochemistry Technology Co. Ltd. China), ozone reactor column (600mm in height and 40mm in diameter) of 0.7L volume, and a peristaltic pump (LEAD-1, Baoding Lange Pump Co. Ltd., China) for feeding the model water into the reactor. A schematic diagram of the ozonation system is shown in Fig. 1. Ozone was produced from an ordinary grade air and fed into the ozone contact column through a porous glass frit at the base of the column, which enables small bubbles to be produced. The residual ozone in the off-gas was adsorbed by KI solution and analyzed by using the standard method.
Before conducting the test, the catalyst was put into the reaction column. The experiment was performed in continuous (catalytic ozonation process) or semicontinuous (ozonation process) flow mode. In the continuous flow mode the flow rate of water is 0.075 L/min, the hydraulic retention time (HRT) was 8 min. In the case of ozonation alone, 0.6L of model water was fed into the reactor column first and ozonated for 8 min, and then the samples were taken out through the outlet. The oxidation reaction was quenched by adding a small amount of sodium thiosulphate. In the adsorption test, air was fed into the reactor column under the same operation condition with ozonation. The test of ozone decomposition was carried out as follows: Firstly, ozone was introduced into a 0.6L distilled water without pH adjustment, then a certain amount of catalyst was added rapidly, and the pH was adjusted by perchloric acid or NaOH. At the same time the magnetic stirrer was switched and the samples were withdrawn at different intervals to determine the residual ozone concentration.
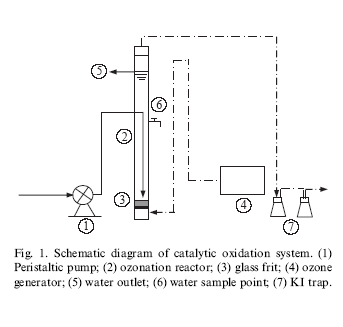
2.4. Analysis
Concentration of nitrobenzene was analyzed by using a SP-502 Gas Chromatograph (GC) equipped with an electron capture detector (Lu Nan Instrumental Factory,Shandong, China). The GC was connected with an on-line GCworkstation, which recorded the data and GCspectra. The chromatographic conditions were as follows: column temperature 160 1C; detector temperature 200 1C; injector temperature 200 1C; carrier gas was nitrogen. The pH was recorded by pHS-2Canalyzer (Lei Ci Instrumental Factory, Shanghai, China). The ozone concentration in the liquid was determined by the Karman-Indigo method (Bader and Hoigne?, 1981).
3. Results
As mentioned above, in a heterogeneous catalytic ozonation system, pH has two main direct influences on the oxidation, i.e., ozone decomposition and the surface property of solid catalyst. On one hand, it is well known that ozone decomposition usually has a high dependence on pH. As shown in Fig. 2, ozone decomposition into active species is catalyzed by OH ion, and the primary radicals such as HOd is formed, which reacts with the organics in a non-selective mode and exhibits high reaction rate constants with the organics (Staehelin and Hoigne?, 1982). It is also reported that OH is a rather poor initiator of aqueous phase radical chain reaction that accelerates the transformation of ozone. It is reported that OH ion exhibits an especially low reaction-rate constant (k ?70 7M1 s1) with ozone, and is only relevant in very pure water (Staehelin and Hoigne?, 1982). Ozone decomposition is also affected by pH, which is related to the equilibrium concentrations of the
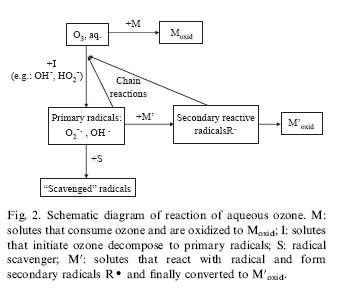
dissociated forms of inorganic carbons in aqueous matrix (HCO3/CO3 2, pKa (HCO3/CO3 2) ?10.3). As shown in Eqs. (1) and (2), carbonate is a much stronger scavenger than bicarbonate. HCO3 ?HOd !H2O ?CO3 d; k ?1:5 107LM1 s1餒oign 磂 and Bader; 1976? (1)
CO3 ?HOd! OH ?CO3 d; k ?4:2 108LM1 s1餗asten and Hoign 磂; 1992?
(2) On the other hand, in heterogeneous catalytic system, pH could affect the surface property of metal oxides (MnOx), which are covered by surface hydroxyl groups in the presence of water. Under different pH conditions, there exists proton transference on the surface of metal
oxides (Stumm and Morgan, 1981). It is shown in Eqs. (3) and (4) that with the increase of pH, the
MeOH2
decreases and more MeO are produced. These species have associations with the adsorption of organics on the catalyst (Liu and Tang, 2000). Up till now, no information has been obtained about the specific interactions of ozone with these species on the surface of metal oxides, but it could be deduced that these species may play an important role for ozone decomposition in heterogeneous catalytic process. RMeOH?
RMeOH ?H? (3) RMeOH MeO ?H? (4)
3.1. Effect of pH on ozonation
The effect of pH on ozonation was studied in semicontinuous mode. As shown in Fig. 3, in acidic and weakly acidic conditions, the ozone molecule itself was one of the main reactive species, so the degradation of nitrobenzene exhibited low efficiency. After the pH was
increased over 6.40, especially higher than 8.30, the degradation efficiency of nitrobenzene began to rise obviously. When pH increased from 8.30 to 10.30, the degradation efficiency increased from 30% to 86%. This was due to the OH ions that functioned as the initiator of radical chain reaction to form hydroxyl radicals, which have high reaction reactivity towards nitrobenzene (k ?2:2 108M1 s1; Hoigne?and Bader, 1976). From Fig. 3, it was also noted that the optimum pH for ozone oxidation of nitrobenzene is 10.30 in this test. At much higher pH, the degradation efficiency decreased. The negative effect should be due to the scavenging potential of carbonate or the interactions between hydroxyl radicals (Gottschalk et al., 2000). Because the solution was prepared with distilled water, the concentration of inorganic carbon was low and the inhibiting impact of carbonate on the oxidation could be
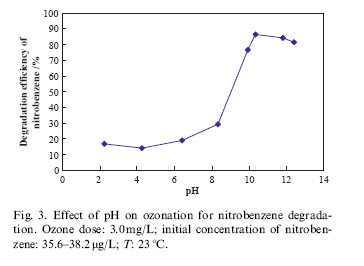
3.2. Effect of pH on MnOx/GAC catalyzed ozonation
The tests were originally planned and carried out in semicontinuous flow mode. However, it was found that except for the initial pH which was adjusted in strong acid region (o3), the pH in effluent (pH about 9) was much higher than that of the influent. In catalyst preparation process, such reaction occurs:
MnO4 t C t H2O !MnOx t CO t OH: (6)
These OH ions may be physically adsorbed on the surface of the catalyst, and they are also easily desorbed into the solution. Whether these adsorbed and desorbed OH ions contribute to the catalyst activity is very interesting to investigate. So the test was planned to
measure the effluent pH continuously. Also, for the heterogeneous catalytic ozonation, long-time continuous tests could give a deeper understanding of pH influence on MnOx/GACcatalyzed ozonation. In the tests, the solution with influent pH 2.65 was adjusted by perchloric acid, and the solution with influent pH 5.98 was not adjusted with acid or base. The experimental results are shown in Tables 2 and 3
and Fig. 4. From Table 2, it is seen that when the influent pH is 2.65, the effluent pH values were slightly changed during the catalytic reaction (2.74–3.52). When the influent pH is 5.98, as illustrated in Table 3, within the initial 15 min of the reaction, the pH of the effluent increased from 5.98 to 9.51–9.61. As the reaction went on, the pH in the effluent gradually decreased. When the reaction time was extended up to 230 min, the effluent.
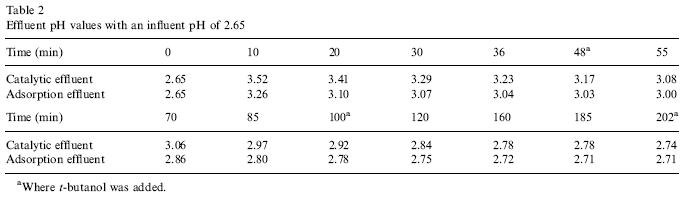
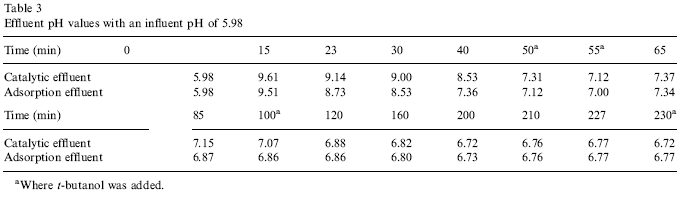
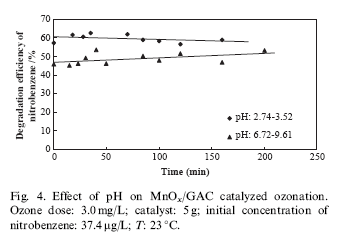
pH decreased to about 6.72. In contrast to this pH change, it was found from Fig. 4 that the degradation efficiency of nitrobenzene did not change with the variation of pH in the solution. From the experimental results, it was expected that in MnOx/GACheterogeneous catalytic ozonation, the surface property of metal oxides, rather than OH ions in the solution, was the dominating factor influencing the catalytic activity. As shown in Fig. 4, it was obvious that in catalytic ozonation, the solutions with pH 2.74–3.52 (acidic condition) had much higher degradation effectiveness of nitrobenzene than the solutions with pH 6.72–9.61 (neutral pH and weak basic condition). Under acidic
condition (pH 2.74–3.52), the degradation efficiency of nitrobenzene was as high as 60%, while in the solutions with pH 6.72–9.61, the degradation efficiency of nitrobenzene was about 50%. Though the tests of the effect of pH on ozonation and catalytic ozonation were performed in different modes (semicontinuous and continuous modes), by comparing the results shown in Figs. 3 and 4, consistent with
previous research results (Ma and Sui, 2002), the MnOx/ GACcatalytic ozonation process is superior to ozonation for the micropollutants degradation.
4. Discussion
In the catalytic ozonation, the understanding of how solid catalyst influences ozone decomposition and the organics adsorption in different pH ranges is helpful for studying the reaction mechanism. As shown in Fig. 5, the presence of MnOx/GACobviously accelerated the decomposition of ozone, and the ozone decomposed faster in pH 5.60 than in pH 3.60. It is also noted that in the presence of catalyst, the decomposition rates at pH
3.60 and 5.60 are similar to the case when pH is 9.10 in the absence of catalyst. Whether hydroxyl radical is also produced in heterogeneous catalytic ozonation is the key
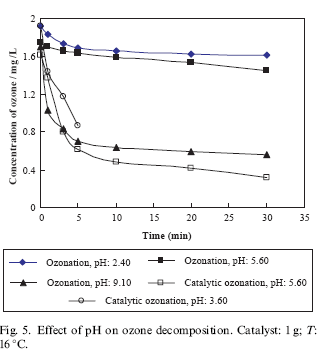
point for understanding the reaction mechanism. Until now, no clear conclusions have been drawn out on catalytic oxidation mechanism. On one hand, though having different postulations about how the initiation processes take place, a majority of authors assumed that the generation of hydroxyl radical due to ozone decomposition at the solid surface was mainly responsible for the improvement of catalytic ozonation (Ma and Graham, 1999; Cooper and Burch, 1999; Lima Hyung-Nam et al., 2002). Some investigators had different views, for they found that the presence of bicarbonate did not have any impact on some heterogeneous catalytic ozonation processes (Paillard et al.,
1991; Logemann and Annee, 1997). In order to evaluate if the MnOx/GACcatalyzed ozonation follows the hydroxyl radical reaction mechanism,
the effect of TBA on the catalytic oxidation was investigated. It is known that TBA is a strong hydroxyl radical scavenger which has the reaction rate constant of 6108M1 s1 with HOd (Buxton et al., 1988) and 3103M1 s1 with ozone (Hoigne′ and Bader, 1983). The TBA was added at 48, 100, and 202 min in influent pH 2.65, and 50, 55,100, 230 min in solutions with an influent pH of 5.98. If the catalytic oxidation follows hydroxyl radical reaction mechanism, the presence of 25 mg/L TBA would rapidly consume HOd?and have a negative impact on the degradation of nitrobenzene.Fig. 6 illustrates the effect of TBA on the oxidation of nitrobenzene by ozone alone in pH 8.32 and 11.80. It was seen that the addition of TBA obviously inhibited the degradation of nitrobenzene. However, it is shown in Figs. 7 and 8 that TBA did not have any inhibition on catalytic ozonation under the investigated pH conditions; the degradation efficiency of nitrobenzene was not
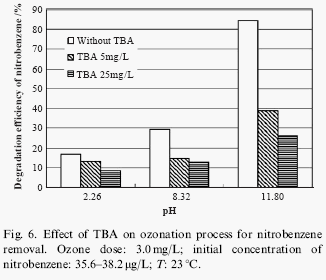
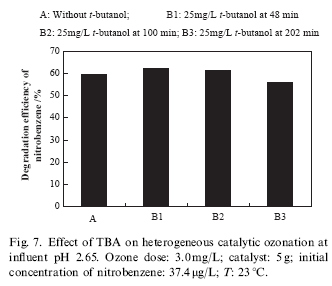
influenced in the presence of tert-butanol. From the experimental results, it may be assumed that hydroxyl radicals might not be the dominating active species in the catalytic MnOx/GACozonation process. The effect of pH on the adsorption of nitrobenzene is shown in Fig. 9. Under the two investigated influent pH values, the difference of adsorption efficiency of nitrobenzene was about 20%. It is obvious that low pH condition benefited for the adsorption of nitrobenzene on the catalyst. This result was consistent with the effect of pH on the catalytic ozonation. Then another question about the role of the catalyst is brought forward. Does the catalyst act more like an adsorbent
in the heterogeneous catalytic process? To clarify this question, continuous flow tests for longer time (hundreds of hours) were performed to compare the different degradation effectiveness of catalytic ozonation and adsorption. It is observed from Fig. 10 that, with the
extension of operation time, the adsorption efficiency of
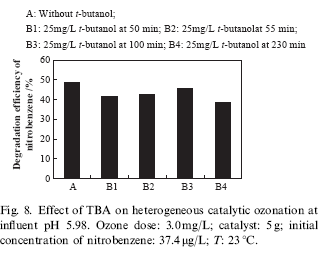
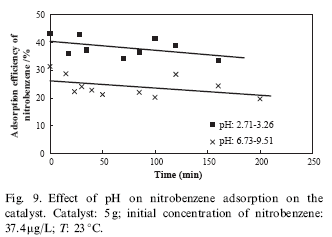
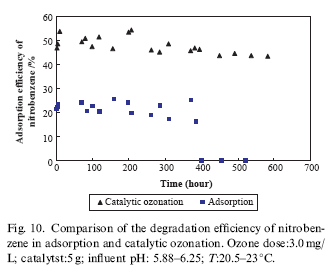
nitrobenzene on MnOx/GACdecreased, and finally in about 400 h, the catalyst reached its adsorption capacity.MnOx/GACcatalyzed ozonation exhibited high degradation efficiency for nitrobenzene in the continuous tests. From the results it is not difficult to infer that in
MnOx/GACcatalyzed ozonation, the adsorption of organic micropollutants on catalyst is an important step, which would have direct influence on the effectiveness of heterogeneous catalytic oxidation; and the organic pollutants may be further decomposed on the surface
of catalyst.
5. Conclusions
Continuous flow experiments were performed to study the effect of pH on MnOx/GACcatalyzed ozonation, and the following conclusions could be made:
1. In the MnOx/GACcatalyzed ozonation, the oxidation efficiency of nitrobenzene was higher at low pH (pH 2.74–3.52) than that at high pH conditions (6.72–9.61). This result was different from the case of ozone oxidation alone.
2. In the presence of MnOx /GACcatalyst, ozone decomposition was accelerated, and higher pH was beneficial to ozone decomposition. It is assumed that hydroxyl radicals might not be the dominating active species in the catalytic oxidation, for the presence of t -butanol had less influence on MnOx/GACcatalyzed ozonation.
3. In MnOx /GACcatalyzed ozonation, the adsorption of organic micropollutants on catalyst was an important step, which would have a direct influence on the catalytic effectiveness of the heterogeneous catalytic system; it is also assumed that the organic pollutants would be further decomposed on the surface of catalyst. Acknowledgements The support from the National Natural Science Foundation of China (NSFC, Project 50378028) is greatly appreciated.
References
Al-Hayek, N., Legube, B., Dore′ , M., 1989. Fe3+/Al2O3- catalysed ozonation of phenol and its ozonation byproduct. Environ. Technol. Lett. 10, 415–425.
Andreozzi, R., Caprio, V., Insola, A., Marotta, R., Tufano, V., 1996. The use of manganese dioxide as a heterogeneous catalyst for oxalic acid ozonation in aqueous solution. Appl. Catal. A: General 138, 75–81. Andreozzi, R., Caprio, V., Insola, A., Marotta, R., Tufano, V.,
1998. The ozonation of pyruvic acid in aqueous solutions catalyzed by suspended and dissolved manganese. Water Res. 32 (5), 1492–1496.
Andreozzi, R., Marotta, R., Sanchirico, R., 2000. Manganesecatalysed ozonation of glyoxalic acid in aqueous solutions. J. Chem. Biotechnol. 75, 59–65.
Bader, H., Hoigne′ , J., 1981. Determination of ozone in water by the indigo method. Water Res. 15, 449–456. Bhat, N., Gurol, M.D., 1995. Oxidation of chlorobenzene by ozone and heterogeneous catalytic ozonation. 27th Industrial Waste Mid-Atlantic Conference, Bethlehem, PA, USA, July, p. 371.
Buxton, G.V., Greenstock, C.L., Helman, W.P., Ross, W.P., 1988. Critical review of rate constants for reactions of hydrated electrons, hydrogen atoms and hydroxyl radicals in aqueous solution. J. Phys. Chem. Ref. Data 17, 513–886. Cooper, C., Burch, R., 1999. An investigation of catalytic ozonation for the oxidation of halocarbons in drinking water preparation. Water Res. 33, 3695–3700. Gottschalk, C., Libra, J.A., Saupe, A., 2000. Ozoantion of Water and Wastewater. Wiley-VCH Verlag GmbH, D- 69469 Weinheim, p. 13. Hoigne′ , J., Bader, H., 1976. The role of hydroxyl radical reactions in ozonation process in aqueous solutions. Water Res. 10, 377–386.
Hoigne′ , J., Bader, H., 1983. Rate constants of reactions of ozone with organic and inorganic compounds in water-I: non-dissociating organic compounds. Water Res. 17 (2), 173–183.
Lima Hyung-Nam, Choib Hechul, Hwangc Tae-Moon, Kangc Joon-Wun, 2002. Characterization of ozone decomposition in a soil slurry: kinetics and mechanism. Water Res. 36, 219–229.
Liu Ruixia, Tang Hongxiao, 2000. Oxidative decolorization of direct light red f3b dye at natural manganese mineral surface. Water Res. 34 (16), 4029–4035.
Logemann, F.P., Annee, J.H.J., 1997. Water treatment with a fixed bed catalytic ozonation process. Water Sci. Technol. 35, 353–360.
Ma, J., Graham, N.J.D., 1997. Preliminary investigation of manganese-catalyzed ozonation for the destruction of atrazine. Ozone Sci. Eng. 19 (3), 227–240.
Ma, J., Graham, N.J.D., 1999. Degradation of atrazine by manganese-catalysed ozonation—influence of humic substances. Water Res. 33 (3), 785–793.
Ma, J., Graham, N.J.D., 2000. Degradation of atrazine by manganese catalysed ozonation—influence of radical scavengers. Water Res. 34 (15), 3822–3828.
Ma, J., Sui, M. H., 2002. Degradation of refractory organic pollutants by catalytic ozonation. Mn-modified activated carbon as catalyst. Advances in Ozone Science and Engineering: Environmental Processes and Technological Applications. 15–16 April, Hongkong, 185–192.
Ma, J., Sui, M.H., Chen, Z.L., Wang, L.N., 2004. Degradation of refractory organic pollutants by catalytic ozonationactivated carbon and Mn-loaded activated carbon as catalysts. Ozone Sci. Eng. 1, 1–9.
Masten, S.J., Hoigne′ , J., 1992. Comparison of ozone and hydroxyl radicals-induced oxidation of chlorinated hydrocarbons
in water. Ozone Sci. Eng. 14, 197–214.
Mullet, M., Fievet, P., Szymezyk, A., Foissy, A., Reggiani, F.C., Pagetti, J., 1999. A simple and accurate determination of the point of zero charge of ceramic membranes. Desalination 121, 41–48.
Paillard, H., Dore′ , M., Bourbigot, M.M., 1991. Prospects concerning applications of catalytic ozonation in drinking water treatment. The 10th Ozone World Congress Proceedings, Monaco, March, pp. 313–329.
Staehelin, J., Hoigne′ , J., 1982. Decomposition of ozone in water: rate of initiation by hydroxide ions and hydrogen peroxide. Environ. Sci. Technol. 16, 676–681.
Stumm, W., Morgan, J.J., 1981. Aquatic chemistry: chemical equilibria and rates in natural waters, 3rd ed. Wiley, NewYork, pp. 516–608.
论文搜索
月热点论文
论文投稿
很多时候您的文章总是无缘变成铅字。研究做到关键时,试验有了起色时,是不是想和同行探讨一下,工作中有了心得,您是不是很想与人分享,那么不要只是默默工作了,写下来吧!投稿时,请以附件形式发至 paper@h2o-china.com ,请注明论文投稿。一旦采用,我们会为您增加100枚金币。